It has been roughly 1 year since Dr Jon Kindem and I finished at Caltech (JK graduating with a PhD and myself – JB – graduating from my postdoc to take up a junior faculty position at the University of Sydney). During our three-and-a-half-year overlap in the IQIM we often told each other that we should write something for Quantum Frontiers. As two of the authors of a paper reporting a recent breakthrough for rare-earth ion spin qubits (Nature, 2020), it was now or never. Here we go…
Throughout 2019, telecommunication companies began deploying 5th generation (5G) network infrastructure to allow our wireless communication to be faster, more reliable, and cope with greater capacity. This roll out of 5G technology promises to support up to 10x the number of devices operating with speeds 10x faster than what is possible with 4th generation (4G) networks. If you stop and think about new opportunities 4G networks unlocked for working, shopping, connecting, and more, it is easy to see why some people are excited about the new world 5G networks might offer.
Classical networks like 5G and fiber optic networks (the backbone of the internet) share classical information: streams of bits (zeros and ones) that encode our conversations, tweets, music, podcasts, videos and anything else we communicate through our digital devices. Every improvement in the network hardware (for example an optical switch with less loss or a faster signal router) contributes to big changes in speed and capacity. The bottom line is that with enough advances, the network evolves to the point where things that were previously impossible (like downloading a movie in the late 90s) become instantaneous.
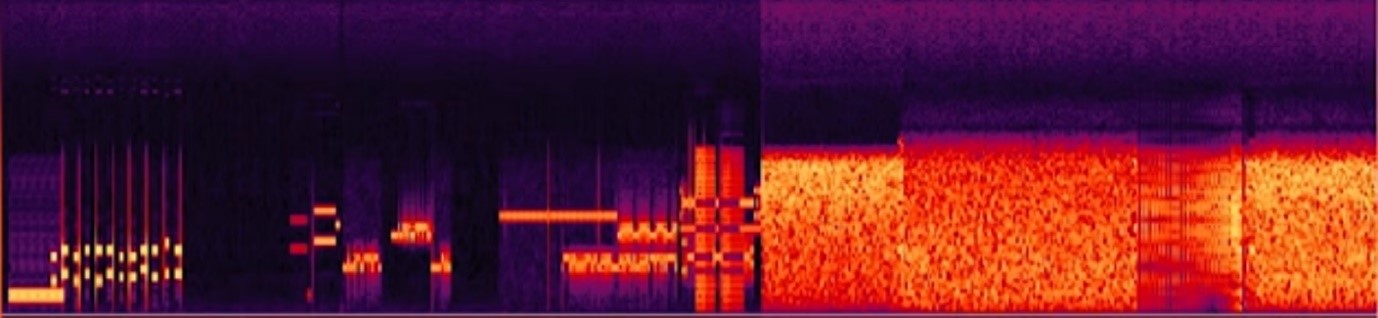
If you are Gen Z or Gen Alpha then you’ll probably need to google dial-up spectrogram.
[Captured from this YouTube video].
Alongside the hype and advertising around 5G networks, we are part of the world-wide effort to develop a fundamentally different network (with a little less advertising, but similar amounts of hype). Rather than being a bigger, better version of 5G, this new network is trying to build a quantum internet: a set of technologies that will allows us to connect and share information at the quantum level. For an insight into the quantum internet origin story, read this post about the pioneering experiments that took place at Caltech in Prof. Jeff Kimble’s group.
Quantum technologies operate using the counter-intuitive phenomena of quantum mechanics like superposition and entanglement. Quantum networks need to distribute this superposition and entanglement between different locations. This is a much harder task than distributing bits in a regular network because quantum information is extremely susceptible to loss and noise. If realized, this quantum internet could enable powerful quantum computing clusters, and create networks of quantum sensors that measure infinitesimally small fluctuations in their environment.
At this point it is worth asking the question:
Does the world really need a quantum internet?
This is an important question because a quantum internet is unlikely to improve any of the most common uses for the classical internet (internet facts and most popular searches).
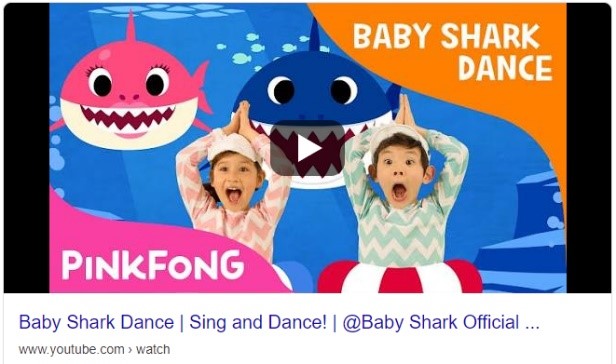
We think there are at least three reasons why a quantum network is important:
- To build better quantum computers. The quantum internet will effectively transform small, isolated quantum processors into one much larger, more powerful computer. This could be a big boost in the race to scale-up quantum computing.
- To build quantum-encrypted communication networks. The ability of quantum technology to make or break encryption is one of the earliest reasons why quantum technology was funded. A fully-fledged quantum computer should be very efficient at hacking commonly used encryption protocols, while ideal quantum encryption provides the basis for communications secured by the fundamental properties of physics.
- To push the boundaries of quantum physics and measurement sensitivity by increasing the length scale and complexity of entangled systems. The quantum internet can help turn thought experiments into real experiments.
The next question is: How do we build a quantum internet?
The starting point for most long-distance quantum network strategies is to base them on the state-of-the-art technology for current classical networks: sending information using light. (But that doesn’t rule out microwave networks for local area networks, as recent work from ETH Zurich has shown).
The technology that drives quantum networks is a set of interfaces that connect matter systems (like atoms) to photons at a quantum level. These interfaces need to efficiently exchange quantum information between matter and light, and the matter part needs to be able to store the information for a time that is much longer than the time it takes for the light to get to its destination in the network. We also need to be able to entangle the quantum matter systems to connect network links, and to process quantum information for error correction. This is a significant challenge that requires novel materials and unparalleled control of light to ultimately succeed.
State-of-the-art quantum networks are still elementary links compared to the complexity and scale of modern telecommunication. One of the most advanced platforms that has demonstrated a quantum network link consists of two atomic defects in diamonds separated by 1.3 km. The defects act as the quantum light-matter interface allowing quantum information to be shared between the two remote devices. But these defects in diamond currently have limitations that prohibit the expansion of such a network. The central challenge is finding defects/emitters that are stable and robust to environmental fluctuations, while simultaneously efficiently connecting with light. While these emitters don’t have to be in solids, the allure of a scalable solid-state fabrication process akin to today’s semiconductor industry for integrated circuits is very appealing. This has motivated the research and development of a range of quantum light-matter interfaces in solids (for example, see recent work by Harvard researchers) with the goal of meeting the simultaneous goals of efficiency and stability.
The research group we were a part of at Caltech was Prof. Andrei Faraon’s group, which put forward an appealing alternative to other solid-state technologies. The team uses rare-earth atoms embedded in crystals commonly used for lasers. JK joined as the group’s 3rd graduate student in 2013, while I joined as a postdoc in 2016.
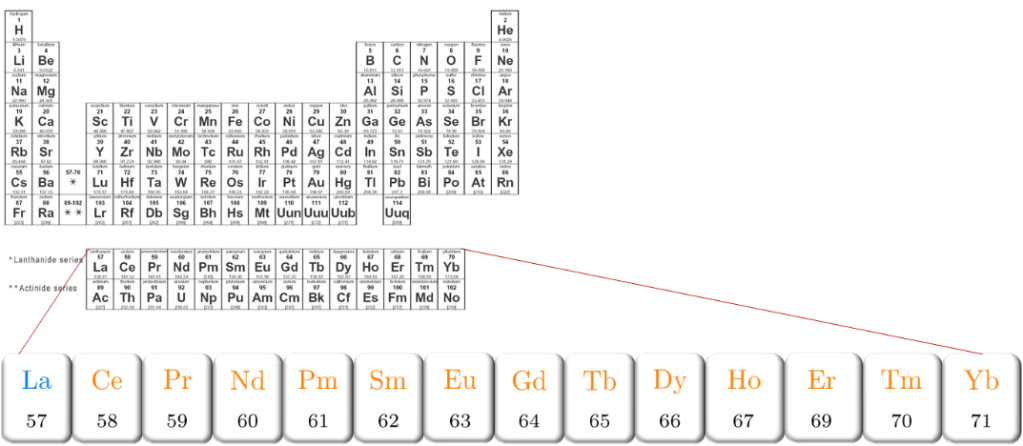
Rare-earth atoms have long been of interest for quantum technologies such as quantum memories for light because they are very stable and are excellent at preserving quantum information. But compared to other emitters, they only interact very weakly with light, which means that one usually needs large crystals with billions of atoms all working in harmony to make useful quantum interfaces. To overcome this problem, research in the Faraon group pioneered coupling these ions to nanoscale optical cavities like these ones:
These microscopic Toblerone-like structures are fabricated directly in the crystal that plays host to the rare-earth atoms. The periodic patterning effectively acts like two mirrors that form an optical cavity to confine light, which enhances the connection between light and the rare-earth atoms. In 2017, our group showed that the improved optical interaction in these cavities can be used to shrink down optical quantum memories by orders of magnitude compared to previous demonstrations, and ones manufactured on-chip.
We have used this nanophotonic platform to open up new avenues for quantum networks based on single rare-earth atoms, a task that previously was exceptionally challenging because these atoms have very low brightness. We have worked with both neodymium and ytterbium atoms embedded in a commercially available laser crystal.
Ytterbium looks particularly promising. Working with Prof. Rufus Cone’s group at Montana State University, we showed that these ytterbium atoms absorb and emit light better than most other rare-earth atoms and that they can store quantum information long enough for extended networks (>10 ms) when cooled down to a few Kelvin (-272 degrees Celsius) [Kindem et al., Physical Review B, 98, 024404 (2018) – link to arXiv version].
By using the nanocavity to improve the brightness of these ytterbium atoms, we have now been able to identify and investigate their properties at the single atom level. We can precisely control the quantum state of the single atoms and measure them with high fidelity – both prerequisites for using these atoms in quantum information technologies. When combined with the long quantum information storage times, our work demonstrates important steps to using this system in a quantum network.
The next milestone is forming an optical link between two individual rare-earth atoms to build an elementary quantum network. This goal is in our sights and we are already working on optimizing the light-matter interface stability and efficiency. A more ambitious milestone is to provide interconnects for other types of qubits – such as superconducting qubits – to join the network. This requires a quantum transducer to convert between microwave signals and light. Rare-earth atoms are promising for transducer technologies (see recent work from the Faraon group), as are a number of other hybrid quantum systems (for example, optomechanical devices like the ones developed in the Painter group at Caltech).
It took roughly 50 years from the first message sent over ARPANET to the roll out of 5G technology.
So, when are we going to see the quantum internet?
The technology and expertise needed to build quantum links between cities are developing rapidly with impressive progress made even between 2018 and 2020. Basic quantum network capabilities will likely be up and running in the next decade, which will be an exciting time for breakthroughs in fundamental and applied quantum science. Using single rare-earth atoms is relatively new, but this technology is also advancing quickly (for example, our ytterbium material was largely unstudied just three years ago). Importantly, the discovery of new materials will continue to be important to push quantum technologies forward.
You can read more about this work in this summary article and this synopsis written by lead author JK (Caltech PhD 2019), or dive into the full paper published in Nature.
J. M. Kindem, A. Ruskuc, J. G. Bartholomew, J. Rochman, Y.-Q. Huan, and A. Faraon. Control and single-shot readout of an ion embedded in a nanophotonic cavity. Nature (2020).
Now is an especially exciting time for our field with the Thompson Lab at Princeton publishing a related paper on single rare-earth atom quantum state detection, in their case using erbium. Check out their article here.
The question do we need a quantum inernet is well answered with those three reasons, but I might suggest another significant sociological reason for needing one Viz., time-technology – which needs perfecting to ensure social sciences progress and deliver humanity from the daily chores of living and getting along frictionfree and implement universal income for all while benefiting with AI to optimize decisions and choices. Here is a link ( https://www.researchgate.net/publication/340662195_The_Public_Benefit_Accounting ) to consider such a need