When I was growing up, one of my favourite places was the shabby all-you-can-eat buffet near our house. We’d walk in, my mom would approach the hostess to explain that, despite my being abnormally large for my age, I qualified for kids-eat-free, and I would peel away to stare at the Koi pond. The display of different fish rolling over one another was bewitching. Ten-year-old me would have been giddy to build my own Koi pond, and now I finally have. However, I built one using Lie algebras.
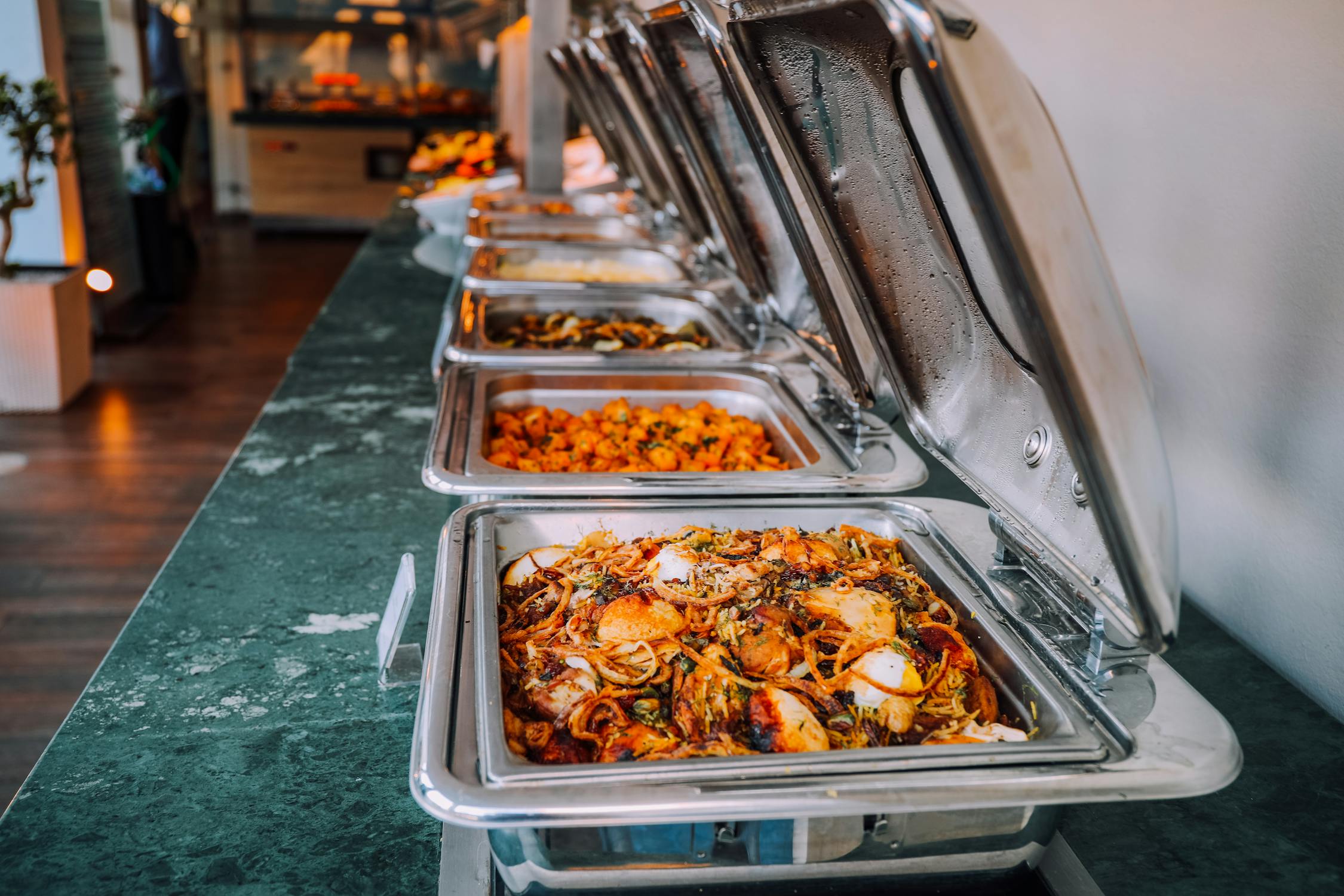
The different fish swimming in the Koi pond are, in many ways, like charges being exchanged between subsystems. A “charge” is any globally conserved quantity. Examples of charges include energy, particles, electric charge, or angular momentum. Consider a system consisting of a cup of coffee in your office. The coffee will dynamically exchange charges with your office in the form of heat energy. Still, the total energy of the coffee and office is conserved (assuming your office walls are really well insulated). In this example, we had one type of charge (heat energy) and two subsystems (coffee and office). Consider now a closed system consisting of many subsystems and many different types of charges. The closed system is like the finite Koi pond with different charges like the different fish species. The charges can move around locally, but the total number of charges is globally fixed, like how the fish swim around but can’t escape the pond. Also, the presence of one type of charge can alter another’s movement, just as a big fish might block a little one’s path.
Unfortunately, the Koi pond analogy reaches its limit when we move to quantum charges. Classically, charges commute. This means that we can simultaneously determine the amount of each charge in our system at each given moment. In quantum mechanics, this isn’t necessarily true. In other words, classically, I can count the number of glossy fish and matt fish. But, in quantum mechanics, I can’t.
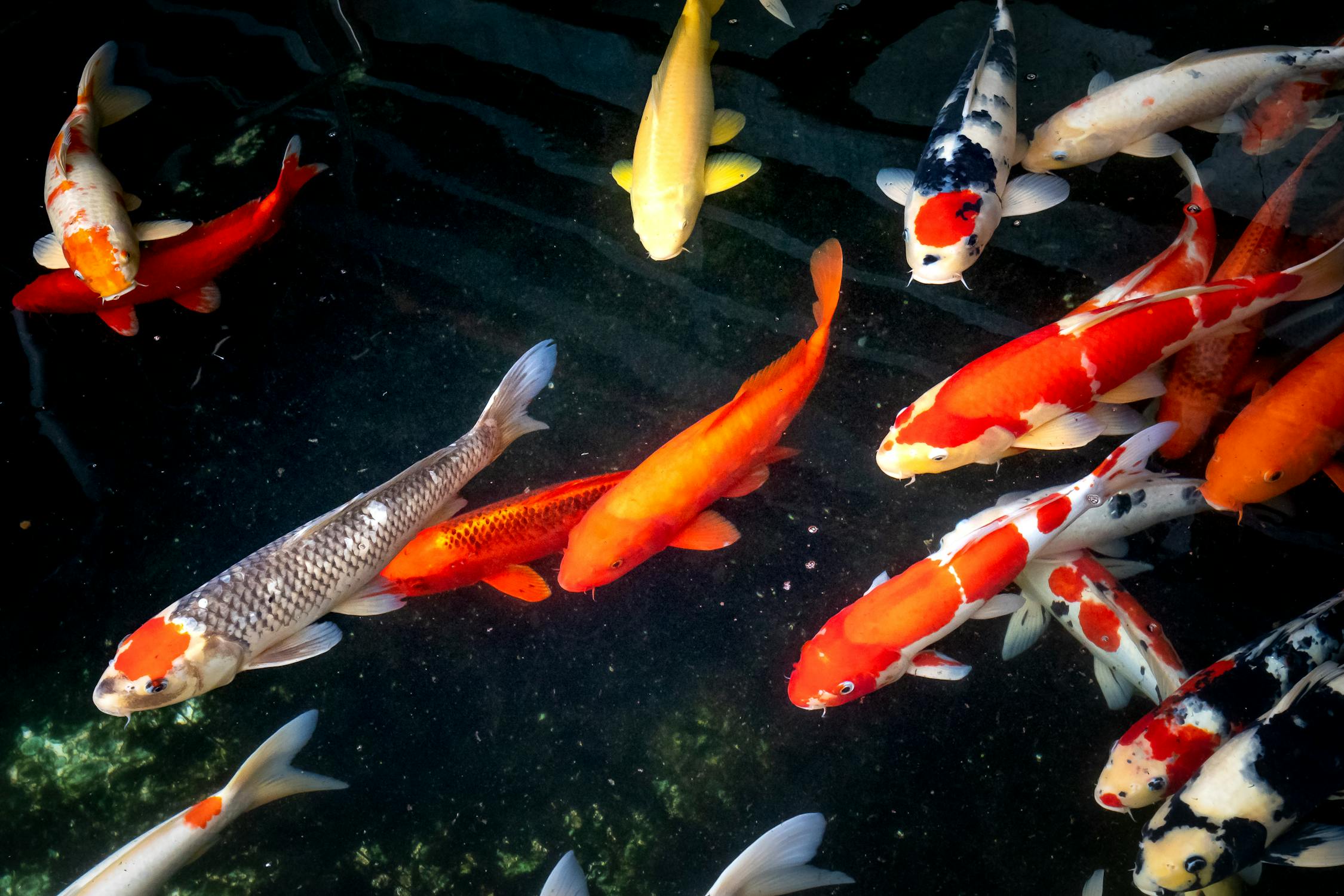
So why does this matter? Subsystems exchanging charges are prevalent in thermodynamics. Quantum thermodynamics extends thermodynamics to include small systems and quantum effects. Noncommutation underlies many important quantum phenomena. Hence, studying the exchange of noncommuting charges is pivotal in understanding quantum thermodynamics. Consequently, noncommuting charges have emerged as a rapidly growing subfield of quantum thermodynamics. Many interesting results have been discovered from no longer assuming that charges commute (such as these). Until recently, most of these discoveries have been theoretical. Bridging these discoveries to experimental reality requires Hamiltonians (functions that tell you how your system evolves in time) that move charges locally but conserve them globally. Last year it was unknown whether these Hamiltonians exist, what they look like generally, how to build them, and for what charges you could find them.
Nicole Yunger Halpern (NIST physicist, my co-advisor, and Quantum Frontiers blogger) and I developed a prescription for building Koi ponds for noncommuting charges. Our prescription allows you to systematically build Hamiltonians that overtly move noncommuting charges between subsystems while conserving the charges globally. These Hamiltonians are built using Lie algebras, abstract mathematical tools that can describe many physical quantities (including everything in the standard model of particle physics and space-time metric). Our results were recently published in npj QI. We hope that our prescription will bolster the efforts to bridge the results of noncommuting charges to experimental reality.
In the end, a little group theory was all I needed for my Koi pond. Maybe I’ll build a treehouse next with calculus or a remote control car with combinatorics.
Pingback: Mo’ heights mo’ challenges – Climbing mount grad school | Quantum Frontiers
Pingback: Mo’ heights mo’ challenges – Climbing mount grad faculty - Veja Online
Pingback: The Noncommuting-Charges World Tour (Part 1 of 4) | Quantum Frontiers